Carbon, the fundamental building block of life and an essential element in countless industrial processes, holds a profound significance across various fields. From its role in shaping the natural world to driving technological advancements, carbon’s versatility is unparalleled. In this article, we delve into the multifaceted nature of carbon, exploring its diverse forms, industrial applications, environmental impact, and future innovations. Join us on a journey to uncover the intricate web of carbon’s influence, and discover why understanding its significance is crucial for shaping a sustainable future.
Carbon in Nature and Biochemistry
Carbon’s ubiquity in nature is nothing short of astonishing. As one of the most abundant elements in the universe, it plays a central role in the formation of molecules essential for life. In biochemistry, carbon’s unique bonding capabilities allow it to form the backbone of organic compounds, including proteins, carbohydrates, lipids, and nucleic acids.
A. Carbon’s Role in Living Organisms:
- Organic Molecules: Carbon’s ability to form stable covalent bonds with other elements, particularly hydrogen, oxygen, nitrogen, and sulfur, enables the creation of an immense variety of organic molecules. These molecules serve as the building blocks of life, participating in essential biological processes.
- Biological Macromolecules: Carbon’s bonding versatility gives rise to complex biological macromolecules such as proteins, which are composed of amino acids linked together by peptide bonds. Additionally, carbohydrates, including sugars and starches, provide energy for cellular processes, while lipids serve as structural components of cell membranes and energy storage molecules.
- Genetic Information: Carbon’s role extends to nucleic acids like DNA and RNA, where it forms the sugar-phosphate backbone that supports the genetic code. The arrangement of carbon atoms within these molecules is critical for encoding and transmitting genetic information across generations.
B. Carbon in the Natural World:
Carbon’s influence extends beyond the realm of biology to shape the natural world in profound ways.
- Carbon Cycle: The carbon cycle describes the movement of carbon through various reservoirs, including the atmosphere, oceans, land, and living organisms. Processes such as photosynthesis, respiration, decomposition, and fossil fuel combustion drive this cycle, regulating Earth’s climate and supporting life.
- Geological Processes: Over millions of years, carbon becomes sequestered in Earth’s crust through geological processes such as sedimentation and mineralization. This carbon reservoir includes fossil fuels like coal, oil, and natural gas, which play a significant role in human civilization as energy sources.
- Climate Regulation: Carbon dioxide (CO2), a byproduct of natural processes and human activities, acts as a greenhouse gas, trapping heat in Earth’s atmosphere and influencing global climate patterns. Understanding carbon’s role in climate regulation is essential for mitigating the effects of climate change.
Industrial and Technological Applications of Carbon
Carbon’s remarkable properties make it indispensable in various industrial processes and technological innovations. From its use as a structural material to its role in energy production and storage, carbon’s versatility drives innovation across diverse sectors.
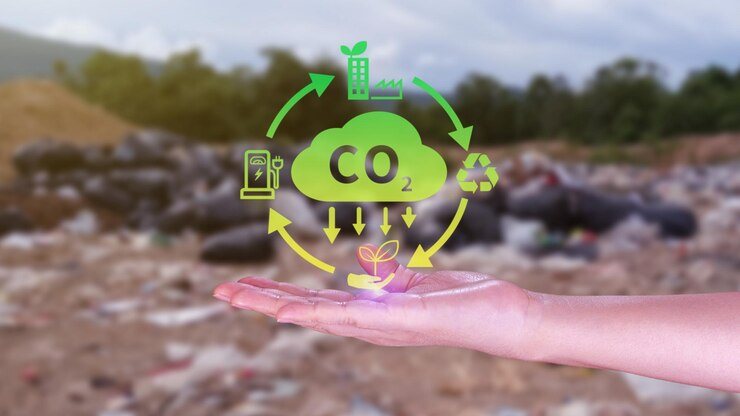
A. Industrial Uses of Carbon:
- Structural Materials: Carbon’s exceptional strength-to-weight ratio makes it an ideal material for structural applications. Carbon fibers, derived from precursor materials such as polyacrylonitrile (PAN) or petroleum pitch, are renowned for their high tensile strength, stiffness, and heat resistance. These properties make carbon fibers invaluable in aerospace, automotive, and sporting goods industries, where lightweight, durable materials are essential.
- Reinforcing Agent: Carbon’s ability to reinforce other materials is exploited in composites manufacturing. Carbon fiber-reinforced polymers (CFRP) combine the strength and rigidity of carbon fibers with the versatility of polymers, resulting in lightweight yet robust materials used in aircraft components, wind turbine blades, and high-performance sporting equipment.
- Carbon Black: Produced by incomplete combustion of hydrocarbons, carbon black is a finely divided form of carbon used as a reinforcing filler in rubber products such as tires, conveyor belts, and hoses. Its high surface area and excellent dispersion properties enhance the mechanical strength and wear resistance of rubber compounds.
B. Carbon in Modern Technology:
- Electronics and Semiconductors: Carbon-based materials play a crucial role in electronics and semiconductor industries. Graphene, a single layer of carbon atoms arranged in a two-dimensional hexagonal lattice, exhibits exceptional electrical conductivity, mechanical strength, and thermal properties. Graphene-based devices hold promise for applications in flexible electronics, transparent conductive films, and high-speed transistors.
- Energy Storage: Carbon materials feature prominently in energy storage technologies, including batteries and supercapacitors. Graphite electrodes, commonly used in lithium-ion batteries, provide a stable platform for intercalation of lithium ions during charge and discharge cycles. Carbon nanotubes and graphene derivatives offer high surface area and electrical conductivity, enabling rapid charge transfer and enhanced energy storage capacity in supercapacitors.
- Catalysis: Carbon-based catalysts play a vital role in chemical synthesis and environmental remediation processes. Carbon-supported metal nanoparticles exhibit catalytic activity in hydrogenation, oxidation, and carbon-carbon bond formation reactions, enabling more efficient and sustainable production of fine chemicals and pharmaceuticals.
Energy Production and Sustainability
Carbon’s impact on energy production and sustainability is multifaceted, encompassing both the challenges posed by carbon emissions and the opportunities presented by renewable energy sources and carbon-neutral technologies. Understanding the interplay between carbon and energy is crucial for addressing climate change and ensuring a sustainable future.
A. Carbon’s Impact on Energy and Sustainability:
- Fossil Fuel Combustion: The combustion of fossil fuels, such as coal, oil, and natural gas, releases carbon dioxide (CO2) and other greenhouse gases into the atmosphere. These emissions contribute to global warming and climate change, posing significant environmental and societal challenges. As the primary source of energy for electricity generation, transportation, and industrial processes, fossil fuels remain central to global energy systems.
- Renewable Energy Sources: In contrast to fossil fuels, renewable energy sources offer a sustainable alternative for meeting global energy demand while reducing carbon emissions. Solar, wind, hydroelectric, and geothermal energy harness natural processes to generate electricity without releasing greenhouse gases. Advancements in renewable energy technologies, such as photovoltaic solar panels, wind turbines, and grid-scale energy storage systems, are driving the transition towards a low-carbon economy.
- Energy Efficiency: Improving energy efficiency is a critical strategy for reducing carbon emissions and enhancing sustainability across various sectors. Energy-efficient technologies, building designs, and transportation systems minimize energy consumption and greenhouse gas emissions while maximizing resource utilization. Investments in energy efficiency initiatives, including energy-efficient appliances, building retrofits, and transportation electrification, yield long-term environmental and economic benefits.
B. Addressing Climate Change:
- Mitigation Strategies: Mitigating the impacts of climate change requires concerted efforts to reduce carbon emissions and transition to renewable energy sources. Policy measures, such as carbon pricing, emissions trading, and renewable energy incentives, incentivize businesses and individuals to adopt low-carbon practices and invest in clean energy solutions. International agreements, such as the Paris Agreement, provide a framework for global cooperation in addressing climate change and limiting global temperature rise.
- Adaptation Measures: In addition to mitigation efforts, adaptation measures are essential for managing the impacts of climate change on vulnerable communities and ecosystems. Adaptation strategies, such as climate-resilient infrastructure, disaster preparedness, and sustainable land management practices, enhance societal resilience and promote long-term sustainability in the face of changing environmental conditions.
- Carbon Capture and Storage (CCS): Carbon capture and storage technologies offer a means of reducing carbon emissions from fossil fuel combustion and industrial processes. CCS involves capturing CO2 emissions at source, such as power plants or industrial facilities, and transporting them to underground storage sites, where they are permanently sequestered in geological formations. While CCS has the potential to significantly reduce carbon emissions, challenges related to cost, scalability, and public acceptance remain barriers to widespread implementation.
Environmental Impact and Management
Carbon’s pervasive presence in the environment has profound implications for ecosystems, biodiversity, and human well-being. Understanding the environmental impact of carbon emissions and implementing effective management strategies are essential for mitigating climate change and promoting environmental sustainability.
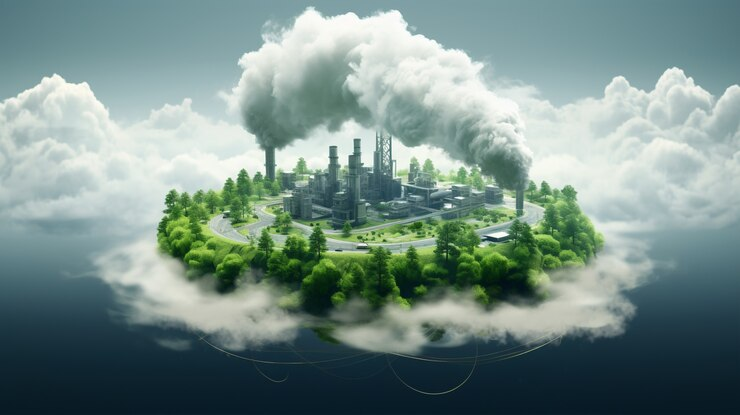
A. Understanding Carbon Footprints:
- Definition: A carbon footprint quantifies the total greenhouse gas emissions, typically measured in carbon dioxide equivalents (CO2e), associated with an individual, organization, product, or activity over a specified time period. It encompasses direct emissions from fossil fuel combustion and industrial processes, as well as indirect emissions from electricity consumption, transportation, and supply chain activities.
- Calculation Methods: Various methodologies exist for calculating carbon footprints, including input-output analysis, process-based life cycle assessment (LCA), and hybrid approaches. These methods consider emissions across the entire lifecycle of products and services, from raw material extraction and production to use and end-of-life disposal.
B. Strategies for Reduction:
- Emission Reduction Targets: Setting ambitious emission reduction targets is a key strategy for mitigating carbon footprints and advancing climate action. Governments, businesses, and individuals can commit to reducing their carbon emissions through initiatives such as renewable energy adoption, energy efficiency improvements, and sustainable transportation choices.
- Transition to Renewable Energy: Transitioning away from fossil fuels towards renewable energy sources is a cornerstone of carbon footprint reduction efforts. Investing in solar, wind, hydroelectric, and geothermal energy infrastructure reduces reliance on carbon-intensive energy sources and promotes a low-carbon energy transition.
- Energy Efficiency Improvements: Improving energy efficiency in buildings, transportation, and industrial processes is another effective strategy for reducing carbon footprints. Energy-efficient technologies, building retrofits, and transportation electrification initiatives help minimize energy consumption and greenhouse gas emissions while enhancing resource efficiency and cost savings.
C. Environmental Impact of Carbon:
- Climate Change: The primary environmental impact of carbon emissions is climate change, characterized by rising global temperatures, shifts in precipitation patterns, and more frequent and severe weather events. Climate change poses significant risks to ecosystems, biodiversity, food security, water resources, and human health, with disproportionate impacts on vulnerable communities and regions.
- Ocean Acidification: Elevated atmospheric CO2 levels contribute to ocean acidification, a process whereby seawater becomes more acidic due to the absorption of CO2. Ocean acidification threatens marine ecosystems, including coral reefs, shellfish populations, and plankton communities, with cascading effects on fisheries, coastal economies, and global food security.
- Air Pollution: In addition to its role in climate change, carbon emissions contribute to air pollution and associated health impacts. Fine particulate matter (PM2.5) and other air pollutants emitted during fossil fuel combustion can cause respiratory illnesses, cardiovascular diseases, and premature death, particularly in urban areas with high levels of pollution.
Economic and Cultural Context of Carbon
Carbon’s significance extends beyond its environmental and technological aspects; it also holds considerable economic and cultural importance. Understanding the economic drivers and cultural perceptions surrounding carbon is essential for shaping sustainable policies and practices.
A. The Economic Aspect of Carbon:
- Energy Markets: Fossil fuels remain dominant in global energy markets, accounting for a significant share of energy consumption and economic activity. Oil, coal, and natural gas extraction, production, and distribution contribute to employment, investment, and revenue generation in energy-producing regions worldwide. However, the economic viability of fossil fuels is increasingly challenged by declining reserves, volatile prices, and environmental concerns.
- Renewable Energy Investments: The transition to renewable energy sources presents significant economic opportunities for investment, innovation, and job creation. Renewable energy technologies, such as solar photovoltaics, wind turbines, and battery storage systems, attract growing investments from governments, businesses, and financial institutions seeking to capitalize on the shift towards a low-carbon economy. Renewable energy industries contribute to economic diversification, energy security, and resilience in the face of climate change impacts.
- Carbon Pricing: Carbon pricing mechanisms, such as carbon taxes and emissions trading schemes, play a crucial role in internalizing the social and environmental costs of carbon emissions. By placing a price on carbon, policymakers incentivize businesses and individuals to reduce emissions, invest in clean technologies, and transition to low-carbon alternatives. Carbon pricing fosters innovation, efficiency, and market competitiveness while generating revenue for climate mitigation and adaptation efforts.
B. Carbon in Cultural and Historical Context:
- Cultural Perceptions: Carbon’s cultural significance varies across societies and historical contexts, reflecting diverse perceptions, values, and traditions. In some cultures, carbon-rich materials like coal and charcoal hold symbolic or ritualistic significance, associated with warmth, sustenance, and transformation. In others, carbon emissions and pollution are sources of environmental degradation, social inequality, and injustice, prompting calls for sustainable development and environmental stewardship.
- Historical Legacy: The industrial revolution and subsequent waves of technological innovation have profoundly shaped human interactions with carbon and its derivatives. The exploitation of fossil fuels for energy and industry has driven economic growth, urbanization, and globalization, transforming landscapes, societies, and economies worldwide. However, this legacy also includes environmental degradation, resource depletion, and social disparities, underscoring the need for more sustainable and equitable approaches to carbon management.
- Cultural Responses to Climate Change: Climate change and its impacts are increasingly recognized as cultural and ethical challenges, requiring collective action and societal transformation. Cultural responses to climate change encompass diverse perspectives, narratives, and creative expressions that emphasize the interconnectedness of humans and nature, the importance of environmental stewardship, and the urgency of climate action. Art, literature, music, and indigenous knowledge systems contribute to climate awareness, resilience-building, and community empowerment in the face of environmental uncertainty.
Future Perspectives and Innovations
The future of carbon holds promise for innovative solutions to pressing global challenges, including climate change, energy security, and sustainable development. Advancements in carbon research and technology are paving the way for novel applications, transformative industries, and a more sustainable future.
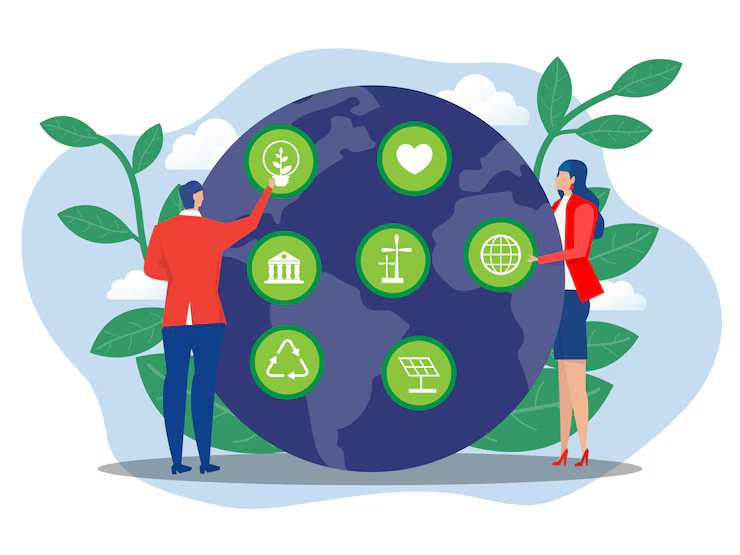
A. Future Innovations in Carbon Use:
- Carbon Capture and Utilization (CCU): Carbon capture and utilization technologies offer a dual benefit of reducing greenhouse gas emissions while creating value-added products. These technologies capture CO2 emissions from industrial sources and convert them into useful materials such as chemicals, fuels, and building materials. By turning CO2 into a resource rather than a waste, CCU holds potential for decarbonizing industrial processes and mitigating climate change.
- Carbon-Negative Technologies: Carbon-negative technologies aim to remove more CO2 from the atmosphere than they emit, effectively reversing the carbon footprint of human activities. Innovations such as direct air capture, enhanced weathering, and bioenergy with carbon capture and storage (BECCS) harness natural or engineered processes to sequester CO2 from the atmosphere or industrial emissions. These technologies have the potential to achieve carbon neutrality and even contribute to carbon removal on a global scale.
- Carbon-Based Materials Engineering: Advances in materials science and engineering are unlocking new possibilities for carbon-based materials with tailored properties and functionalities. Nanomaterials such as carbon nanotubes, graphene, and fullerenes exhibit extraordinary mechanical, electrical, and thermal properties, enabling applications in electronics, energy storage, and structural materials. By designing carbon-based materials at the atomic and molecular levels, researchers are pushing the boundaries of what is possible in fields ranging from medicine to renewable energy.
B. Advancements in Carbon Research:
- Multidisciplinary Approaches: Carbon research is increasingly interdisciplinary, drawing on expertise from fields such as chemistry, physics, biology, materials science, and engineering. Collaborative research efforts facilitate cross-disciplinary innovation, knowledge exchange, and technology transfer, accelerating progress towards sustainable solutions to global challenges.
- Emerging Fields: Emerging fields such as carbon nanotechnology, carbon sequestration, and carbon-neutral technologies are driving innovation and investment in carbon research. These fields offer opportunities for academic research, industrial collaboration, and entrepreneurship, fostering a vibrant ecosystem of innovation and discovery.
- Global Collaboration: International collaboration and partnerships are essential for advancing carbon research and addressing global challenges collaboratively. Initiatives such as the Carbon Sequestration Leadership Forum (CSLF), the Global Carbon Project, and the Mission Innovation initiative bring together governments, research institutions, and industry stakeholders to coordinate research agendas, share best practices, and accelerate the deployment of carbon mitigation technologies.
Conclusion
In closing, the exploration of carbon’s significance across various fields reveals its profound impact on our world and underscores the urgent need for concerted action to address its challenges and opportunities. From its role in driving economic growth and technological innovation to its environmental implications for climate change and sustainability, carbon occupies a central position in shaping our present and future. As we navigate the complexities of carbon management and transition towards a more sustainable and equitable society, the key takeaway lies in the transformative potential of collaboration, innovation, and collective action.
By embracing renewable energy, promoting energy efficiency, advancing carbon capture and utilization technologies, and fostering interdisciplinary collaboration, we can harness the power of carbon to create a more resilient, prosperous, and sustainable world for generations to come. Let our journey through the realms of carbon’s significance serve as a catalyst for meaningful change and inspire us to forge a brighter future for humanity and the planet.
FAQs
1. What is a carbon footprint, and why is it important?
A carbon footprint measures the total greenhouse gas emissions, typically in carbon dioxide equivalents (CO2e), associated with an individual, organization, product, or activity. It is important because it helps quantify the environmental impact of human activities and serves as a tool for identifying opportunities to reduce emissions and mitigate climate change.
2. How can individuals reduce their carbon footprint?
Individuals can reduce their carbon footprint by making lifestyle choices that minimize energy consumption and greenhouse gas emissions. This includes using energy-efficient appliances, reducing meat consumption, using public transportation or carpooling, conserving water, and supporting renewable energy sources such as solar or wind power.
3. What are the benefits of transitioning to renewable energy sources?
Transitioning to renewable energy sources offers numerous benefits, including reducing greenhouse gas emissions and mitigating climate change, enhancing energy security by diversifying energy sources, creating jobs and economic opportunities in the renewable energy sector, and promoting sustainable development and environmental stewardship.
4. How do carbon capture and utilization technologies work?
Carbon capture and utilization (CCU) technologies capture carbon dioxide (CO2) emissions from industrial sources and convert them into useful products, such as fuels, chemicals, and building materials. These technologies typically involve capturing CO2 from flue gases or ambient air using chemical or physical processes, followed by conversion or utilization of the captured CO2 for various applications.
5. What role does carbon pricing play in addressing climate change?
Carbon pricing mechanisms, such as carbon taxes and emissions trading schemes, internalize the social and environmental costs of carbon emissions by placing a price on carbon. This incentivizes businesses and individuals to reduce emissions, invest in clean technologies, and transition to low-carbon alternatives. Carbon pricing helps drive innovation, efficiency, and market competitiveness while generating revenue for climate mitigation and adaptation efforts.